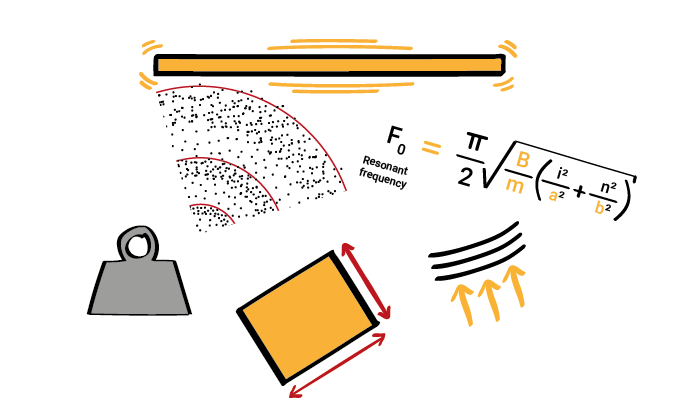
Solid free hanging panels can absorb sound
Did you know that panels, made of a solid and non-porous materials, located freely in the air can absorb sound?
You may not need to know this for all projects requiring acoustic design, but it could be useful if your project involves suspended, free hanging and/or free standing elements like canopies or shells around music stages.
And because it may sound counter intuitive, this short article is here to explain:
- Why solid panels can absorb sound;
- How to specify them;
Enjoy the read!
(See at the end the references used to confirm the concepts)
You want to be notified when new posts are published? Why don’t you subscribe to Atelier Crescendo’s newsletter by clicking here?
Are you looking to find examples of acoustic solutions and products available on the market?
Visit the Acoustic Design Catalogue by clicking on the button below.
Why can solid free hanging panels absorb sound ?
A solid, non-porous panel hanging freely can absorb sound.
However, absorption is minimal at mid and high frequencies (above 500 Hz). It becomes more significant at low frequencies (below 500 Hz).
This is due to ‘mass reactance’.
When sound waves hit a panel, they cause it to move and vibrate, which creates a loss of acoustic energy.
Consequently, panels absorb more energy at low frequencies.
Practically, this happens because larger air particle movements are needed to move a panel.
Note:
You may need to read Fundamental concepts of sounds to understand the relationship between frequencies and the motion of air particules.
How to specify solid free hanging panels?
Obviously, a specification needs to consider all factors that influence a panel’s sound absorption.
One formula estimates the resonant frequency of a simply supported panel:
Note:
Why is it useful to calculate the resonant frequency of a panel?
Because it is the frequency at which a panel moves the most and absorbs the most sound.
with:
- B the bending stiffness of the panel/material;
- m the mass of the panel;
- a and b respectively the width and the length of the panel;
- i and n correspond to the harmonics of resonance, that you don’t need to understand in depth for this article.
This formula may be daunting for you, but you can note the influence of different properties.
Influence of the
mass ‘m’
The higher the mass the lower the resonant frequency.
(And vice versa, the lower the mass the higher the resonant frequency.)
This means that a good specification should reference:
- the material used for the panel(s);
- the density of the material(s);
- the thickness of the panel(s);
- and any other factors influencing the mass of a panels.
Influence of the
stiffness ‘B’
The higher the (bending) stiffness, the higher the resonant frequency.
(And vice versa, the lower the stiffness, the higher the resonant frequency.)
This means that a good specification should reference:
- (again) the material used for the panel(s) ;
- the mounting and fixing conditions of the panel(s);
- and any other factors influencing the mass of the panel(s) like how curved they are (curving a material generally makes it stiffer).
References
See below the references used to confirm the above concepts:
- Architectural Acoustics – Second Edition – Marshall Long – Academic Press
- Acoustic Absorbers and Diffusers – Theory, Design and Application – Third Edition – Trevor Cox, Peter D’Antonio
- Engineering Noise Control – Sixth Edition – CRC Press – David A. Bies, Colin H. Hansen, Carl Q. Howard, Kristy L. Hansen